- 1. Introduction to Neuroethics
- 2. Introduction to Neuroimaging
- 3. Technologies
- 4. Potential uses
- 5. Ethical issues
- 6. Neuroimaging Technology in Popular Culture
- 7. Case Studies
- 8. Conclusion
- 9. References
- Special: Coma to Brain Death: Recognizing Disorders of Consciousness
2. Introduction to Neuroimaging
Few advances have transformed the fields of neuroscience and medicine like brain imaging technology. As the ability to see inside and "read" a living human brain becomes increasingly sophisticated, the ethical and social implications of neuroimaging grow ever greater, shaped by both the capabilities of the technology itself and the public perception of its potential. Beginning in 1919 with the first structural images of the brain by a technique called pneumoencephalography2, the demand for neuroimaging has been unrelenting. But like many other areas of neuroscience, there is a great deal of both hype and hope surrounding neuroimaging. We must consider these often opposing forces as we manage our burgeoning and otherwise unrestricted enthusiasm for these technologies.
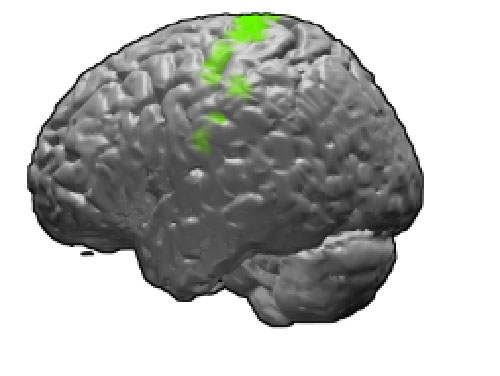
Source: Wikipedia
The ability to "see" the human brain in action, no matter how hazy the image (or the interpretation of that image), is of great significance for modern society. The brain is not just another organ with diseases to be diagnosed and treated through modern medical technology. It holds a special cultural status as the seat of the mind, central to our notions of self and identity.3 As such, images of the brain are filled with far more significance than images of other organs like the lungs or kidneys, and thus requires far more discretion and responsibility for those who produce and make use of such images.
Ethical dilemmas generated by neuroimaging do not just lie in the production of these images. Just as many, if not more, of the dilemmas arise from the limitations in interpreting results and accurately conveying them to the public. When sharing the results of neuroimaging, neuroethicists must address topics such as privacy of unspoken thought and decision-making. Assignment of personal responsibility must be redefined and our concept, of how a "healthy" brain should look and function will be transformed.4
An image has a powerful impact on an unbiased observer, but throughout this discussion, please consider the technical limitations of neuroimaging: there is much yet to learn about the science, and the art, of producing and interpreting images of the brain.
3. Technologies
Neuroimaging utilizes a number of technologies to directly or indirectly produce images of the brain. Each technique is designed to convey distinct types of information, depending on the scientific or medical question at hand.
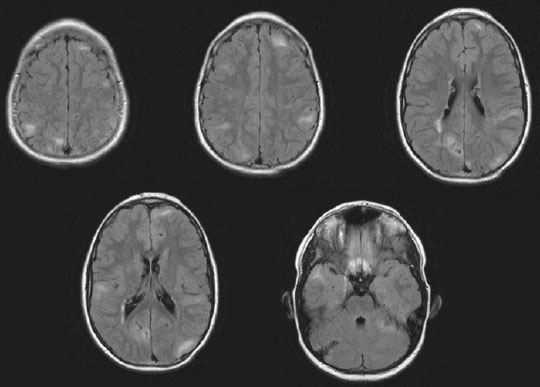
Source: Wikipedia
There are different kinds of neuroimaging. Structural imaging offers a vision of the structure of the brain to enable diagnosis of larger-scale diseases, tumors, injuries, and stroke. Functional imaging is used to diagnose smaller tumors and diseases on a finer scale. Functional imaging also allows us to visualize the relationship between activity in certain brain areas and specific mental functions. Functional imaging is often used in neurological and cognitive science research. Given that most ethical dilemmas addressed in this module pertain to functional imaging, henceforth we will primarily concentrate on the applications and implications of technologies such as fMRI, CT, and PET scans.
3.1. Electroencephalography (EEG)
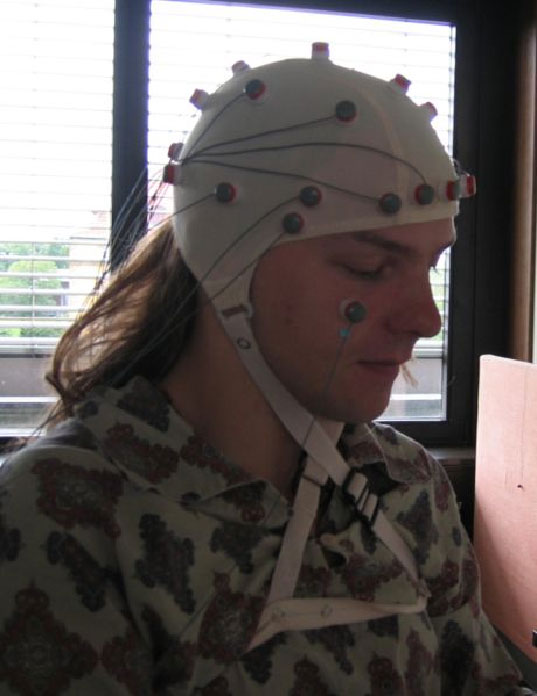
Source: Wikipedia
Multichannel electroencephalography, or EEG, records the electrical activity of the brain via electrodes placed on the scalp. EEG works by recognizing changes and measuring differences in voltage between different parts of the brain (rather than measuring electrical currents directly) and looks for abnormalities in the patterns of activity. The results, or "images," are depicted as a series of wavy lines drawn by a row of pens on a moving piece of paper or as an image on a computer screen. In fact, because of its extremely high temporal resolution, EEG was the first neuroimaging tool to depict the working of the human brain in near real time. 5 EEG has been used routinely for many years, and is a widely-accepted technique featured in a great deal of medical literature. 6
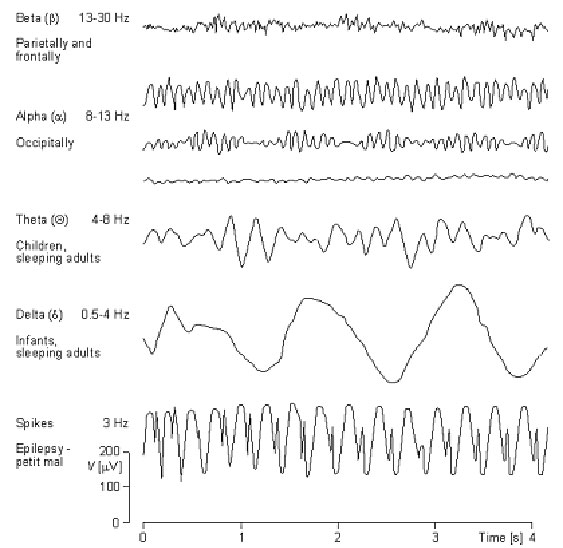
Source: Biogenic
Currently, EEGs are used to distinguish between different types of seizures, diagnose sleep disorders, and to examine head injuries, tumors, infections, and neurodegenerative diseases. EEG can confirm brain death in a comatose patient.7 Much of the benefit of EEG is that it is a non-invasive, safe, and low-cost method of neuroimaging. However, given that it is one of the first types of neuroimaging ever developed (EEG technology dates back to the 1920s), it is considered relatively "primitive" and has limited spatial resolution compared to other techniques.8
3.2. Magnetoencephalography (MEG)
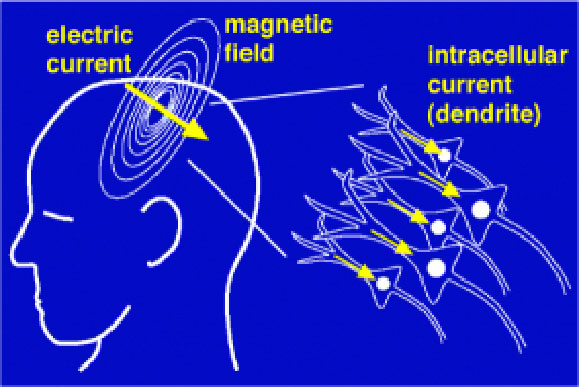
Source: Wikipedia
Magnetoencephalography (MEG), developed during the 1960s, is closely related to EEG, but offers more advanced and accurate imaging. Like EEG, it is a non- invasive and safe technique that gathers data through electrodes placed on the scalp. However, rather than measuring electrical voltage like EEG, MEG measures the magnetic fields produced by electrical currents in the brain. While the skull and the tissue surrounding the brain affect the electrical impulses measured by EEG, they interfere much less in the MEG that measures the magnetic fields. This difference allows for greater accuracy when trying to identify the locations of various brain functions.9 MEG data are often depicted as an outline of the brain showing the directions of the magnetic fields all over it. Regions with unexpected directions that indicate unusual or abnormal activity can be highlighted.10
Clinically, MEG is very useful in locating brain tumors and the sources of epileptic seizures, as well as accurately pinpointing defects in primary auditory, somatosensory, and motor areas. Magnetoencephalography alone is not an efficient diagnostic tool when used during complex cognitive tasks. In these instances, it is often used in combination with other types of imaging technology, particularly fMRIs. In these cases, MEG is used to measure the time courses of brain activity because that cannot be measured with fMRIs. These methods usually compliment one another.
3.3. Computed Tomography (CT)
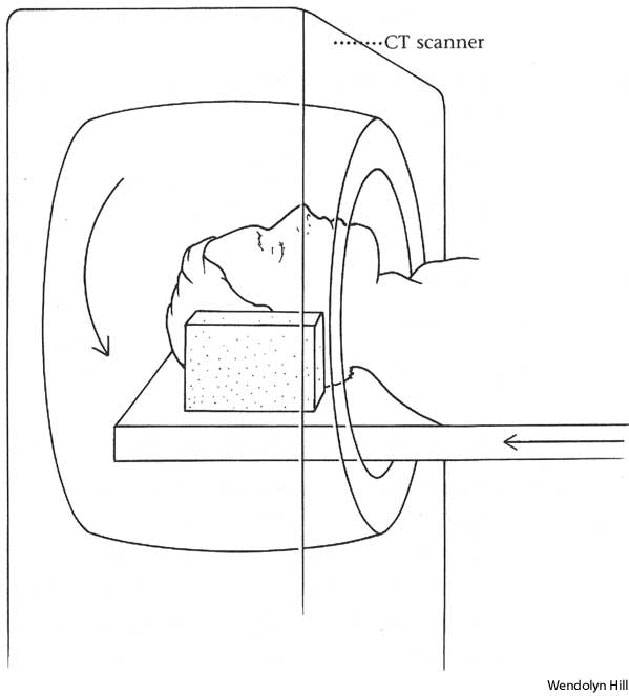
Source: Answers.com
Computed tomography (CT), also known as computed axial tomography (CAT), scanning is a medical imaging method where digital geometry processing is used to generate a three-dimensional image of the interior of an object from a large series of two-dimensional X-ray images taken around a single axis of rotation. Using a CT scan, an X-ray tube rotates around the patient who may have been given a contrasting agent to better define the targeted structures. Advantages of CT technology over X-ray are that it is more sensitive, provides images with higher resolution, and offers more anatomically specific information with greater detail. CT technology is similar to MRI in that they both produce cross-sectional images that appear to open the body up, allowing the doctor to look at it from the inside, but while MRI uses a magnetic field and radio waves to produce images, CT uses X-rays.
The advent of computed tomography (CT) has revolutionized diagnostic radiology. Since its inception in the 1970s, CT use has increased exponentially. It is estimated that in the United States more than 62 million CT scans each year are currently obtained including at least 4 million for children.11 It is considered the gold standard in the diagnosis of many diseases and injuries.
Because of its high-contrast resolution, CT's primary benefit is the ability to separate anatomical structures at different depths within the body. Multiple images are taken during a CT or CAT scan, and a computer compiles them into complete, cross-sectional pictures ("slices") of soft tissue, bone, and blood vessels. The computer then records these slices across the body in a spiral motion. The computer varies the intensity of the X-rays in order to scan each type of tissue with the optimum power. After the patient passes through the machine, the computer combines all the information from each scan to form a detailed image. Because of the high resolution of the images, these scans often result in earlier diagnosis and more successful treatment of many diseases.
CT scans of the head and brain are used to evaluate acute cranial-facial trauma, diagnose acute stroke, check for suspected subarachnoid or intracranial hemorrhage or blood vessel abnormality, as well as to diagnose causes of headaches and loss of sensory or motor function, and to determine if there is an abnormal development of the head and neck.
CT is considered a moderate- to high- radiation diagnostic technique. A typical chest CT delivers a dose of radiation that is approximately 175 times greater than a similar chest X-ray and the equivalent of 20 sets of mammograms. The range of amounts of radiation compared to X-rays of the same body part can be between 20 and 200 times higher depending on which part of the body is being scanned. Direct evidence from epidemiologic studies shows that the organ doses corresponding to a common CT study (two or three scans, resulting in a dose in the range of 30 to 90 mSv) result in an increased risk of cancer. The evidence is reasonably convincing for adults and very convincing for children.12
Because of the ease of use, numbers of scanners available, and its efficacy to rule out injury or disorders, there are concerns that CT scans are being used too frequently -- and perhaps in some cases, unnecessarily. Brenner and Hall caution that the potential carcinogenic effects from using CT scans may be underestimated or overlooked. This is of particular concern, because they claim perhaps one-third of all CT scans performed in the United States may not be medically necessary.13
3.4. Positron emission tomography (PET)
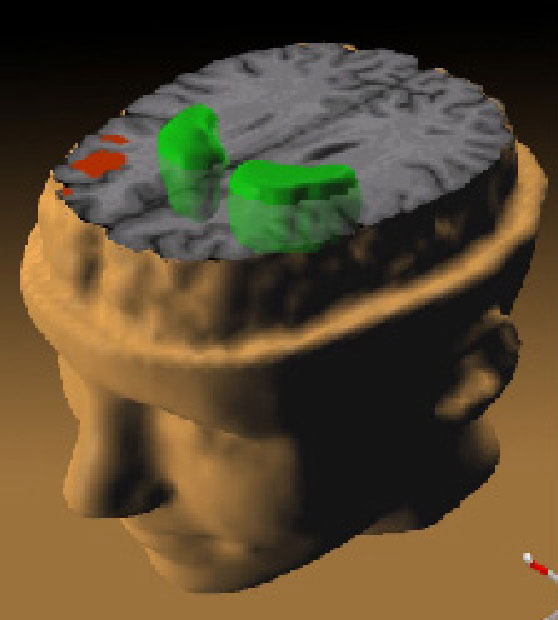
Source: National Institutes of Health
Positron emission tomography (PET) is used to produce a three-dimensional image of how organs and tissues function in the body. In a PET scan, a small dose of a radioactive chemical, a "radiotracer," is injected into the bloodstream, and as the tracer travels through the body, it is absorbed by the organs and tissues being studied. The subject is then scanned with a ring-shaped PET scanner, which looks for the energy given off by the tracer substance. With the aid of a computer, energy emissions are converted into three-dimensional pictures. A PET scan can measure functions like blood flow, oxygen use, and metabolic activity. With this information, doctors can identify abnormalities.14 However, in published figures, PET scans are often mathematical abstractions. They are averaged over many individual subjects, and the exact results differ between individuals.15
Currently, PET scans are most commonly used to detect cancer, heart problems, brain tumors, seizures, and other CNS disorders.16 PET scans will also likely evolve to be used as an imaging tool to predict diseases involving cognitive problems, such as Alzheimer's disease.17 PET scans differ from other imaging technology because they can measure metabolic changes at the cellular level, which usually occur long before any structural changes detected by a CT scan or an MRI.18 However, they are limited in use because of their expense; there are fewer than 100 scanners currently available in the U.S.19
3.5. Single photon emission computed tomography (SPECT)
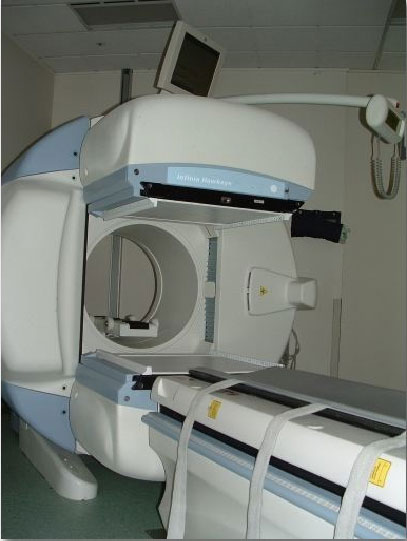
Source: Wikibooks
Single photon emission computed tomography (SPECT) is an imaging technique that uses gamma rays to directly measure blood flow and indirectly measure metabolic activity in the brain. Similar to a PET scan, a radioactive isotope that specifically binds to receptors in the brain is injected into the bloodstream for SPECT imaging.20 The patient is then scanned by a gamma camera to detect where the radioactive isotope is located, and images of the brain are reconstructed based on that information. Multiple 2D images are taken from different angles around the brain, and then a computer program is used to compile the 2D images into a 3D data set.21 Data can actually be reconstructed from almost any angle, which is more flexible than the images produced by imaging techniques such as CT or MRI. SPECT has some advantages to use because it is rapid, portable, and relatively inexpensive.
SPECT has been used to look at psychiatric and neurological disease, including dementia and stroke and seizures disorders, and can also be used to look at damage from head trauma or drug abuse.22 During the 1970s and 1980s, SPECT was almost completely replaced with higher-resolution techniques like CT and MRI, but these two types only offered static images of structure, rather than of dynamic brain activity like SPECT. Over time, SPECT technology has improved vastly to show higher resolution images from deeper within the brain, and is now more commonly in use.23
3.6. Functional magnetic resonance imaging (fMRI)
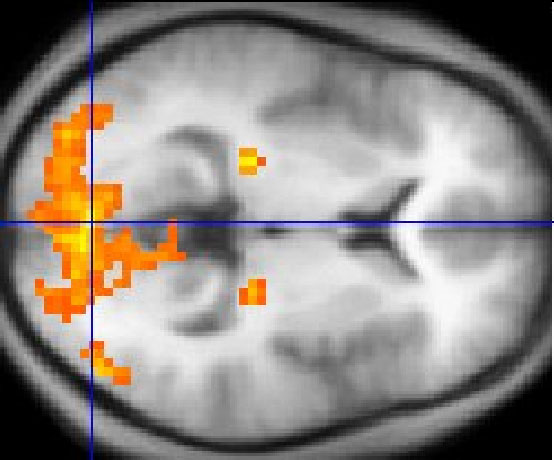
Source: Wikipedia
Functional magnetic resonance imaging (fMRI) is a neuroimaging technique that creates activation "maps" to show which parts of the brain are involved in a particular mental process. When a particular brain area is more active, it consumes more oxygen, and to meet this increased demand, oxygenated blood flow increases to the active area. Because oxygenated and deoxygenated blood interact differently with magnetic fields, activity in the brain can be detected using a scanner with a magnetic field.24 Data can appear as a detailed image of the brain, highlighting the regions that are active during a certain mental function, and multiple images can be taken over time to show change throughout a specific period of time. The amount of change in oxygenated blood flow can also be determined.25
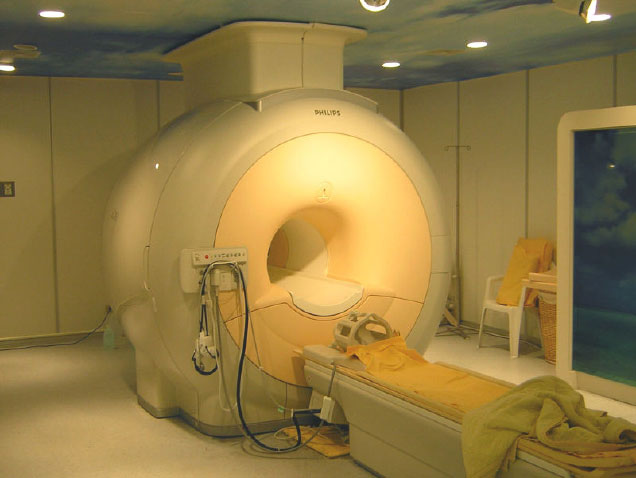
Source: Wikimedia
Functional MRI offers several advantages over other types of neuroimaging. Most important is that it does not use radioactive isotopes, thus making it safer for the patient. It is non-invasive, has excellent image resolution, and is relatively easy to use.26 In fact, fMRI stands out as the neuroimaging technology most likely to have the greatest impact on our society, both inside and outside research and medicine.27
Functional MRI has become a very popular technique for imaging normal brain function and providing information on diverse topics of study, such as memory formation, language, pain, learning, emotion, and decision-making, among many others.28 In most published papers, as with PET scans, figures are created by averaging the resulting image among many patients, rather than taking data from a single patient.29 In the clinical setting, fMRI has a small but growing role: it is used in pre-surgical planning to localize brain function; there is potential for it to be used in presymptomatic diagnosis and for understanding certain brain disorders; and it is also used to study chronic pain.30 Superimposed patterns of neural activity as estimated from blood flow on static high resolution structural images have helped define a new functional anatomy.
4. Potential uses
Neuroimaging technology has not yet realized its full potential. While the use of these techniques in research and clinical settings has increased exponentially within the past decade, there are a burgeoning set of applications that seem to deviate from the more common medical and scientific uses. In this section, we will address some of the common, as well as the more unusual and spectacular uses of neuroimaging, many of which are still in the early stages of development.
4.1. States of consciousness
Currently, all three major disorders of consciousness--coma, vegetative state, and minimally conscious state--are diagnosed by clinical observation and a neurological examination. While diagnoses are sometimes supported by structural neuroimaging that show damage consistent with the clinically observed state, neither the diagnosis nor prognosis for any of the three states of consciousness actually depends on or includes the results of structural or functional neuroimaging.
Disorders of consciousness involve vast numbers of patients and resources. The 1994 estimates cited 10,000 to 25,000 adults and 4,000 to 10,000 children then living in a persistent vegetative state.31,32 Hirsch cited an estimated 15,000 vegetative patients in the US, with an additional 100,000 being minimally conscious.33 These papers all note the paucity of epidemiological research on the populations of minimally conscious persons, a fact that has hampered research. In 1994, a rough approximation of the total annual costs in the United States for the care of adults and children in a persistent vegetative state was $1 billion to $7 billion.34 Estimates on prevalence are inexact, as there is insufficient epidemiologic data on this population. Advances in neurocritical care may alter the statistics, but it is not clear in which direction. On one hand, more patients who might previously have died now survive only to endure years of severe disability. Conversely, advances such as cooling after cardiac arrest stand to greatly decrease the numbers of patients who previously would have been left with devastating brain injury.35
Please click here for Coma to Brain Death, an in-depth discussion of states of consciousness, their causes, their clinical manifestations, and their treatments.
4.1.1. Neuroimaging and Disorders of Consciousness
Discussions surrounding the interface between neuroimaging and disorders of consciousness center on two main issues: the accuracy of diagnosis of vegetative versus minimally conscious state--specifically whether patients thought clinically to be completely unaware truly are--and the possibility of late recovery, specifically whether functional neuroimaging could contribute to predicting this rare occurrence.
A noteworthy feature of the vegetative state and minimally conscious state criteria is that they are clinical and do not include imaging studies. Earlier definitions, and the work of the Multi-Society Task Force in particular, also excluded imaging from diagnosis. Nevertheless they included it as a future direction, suggesting that PET and SPECT studies could help to decide which patients were in a vegetative state--rather undermining the clinical diagnosis they had just defined--and to confirm that these patients lacked awareness.
Currently, all three major disorders of consciousness -- coma, vegetative state, and minimally conscious state -- remain clinical diagnoses rooted in the neurological examination and often are supported by structural imaging showing damage consistent with the clinically observed state. At present, prognosis is based on the established data, and although imaging is frequently performed in the course of formulating a diagnosis for these patients, neither diagnosis of nor prognosis for any of the three states depends on or includes the results of structural or functional neuroimaging.
At the research level, however, functional and metabolic neuroimaging are enhancing scientists' understanding of disordered consciousness. Their roles are manifold. For one, technologies such as PET can provide neuroanatomical information about which brain regions are affected after brain injury. Such metabolic studies deepen our understanding of the physiologic state of injured tissue. They have bolstered clinical diagnoses by showing decreased metabolism commensurate with the observed neurological exam in patients with severely disordered consciousness. For example, scans from clinically brain dead patients show no metabolism, while those from comatose patients show globally or regionally reduced cortical metabolism similar to that of patients under anesthesia.
Brain scans of vegetative patients show injured primary and association cortex metabolizing at 30-40% of normal and sparing brain stem structures, consistent with these patients' "awake but unaware" status. Scans also demonstrate damage to connections between receptive and processing areas consistent with these patients' lack of interactivity. Minimally conscious patients' scans show lower levels of reduced metabolism in the affected areas, differences in the medial parietal and posterior cingulate, and larger areas of preserved function and connectivity compared to vegetative patients' scans.36 In some cases, these patients' brains are less active than normal at rest, but demonstrate activation of larger neural networks when given auditory stimuli.37
4.1.2. Accuracy of diagnosis
In terms of diagnosis, at least one study has shown that diagnostic concordance among physicians distinguishing clinically between the vegetative and minimally conscious states is imperfect, even among neurologists.38 Since the distinction would lead to prognostic statements for those patients diagnosed with vegetative state, in turn prompting decisions about continuing life support and/or artificial nutrition and hydration that could ultimately result in death, there is a need to diagnose these patients as accurately as possible.
Beyond accuracy of clinical diagnosis, neuroimaging technology has raised new questions of whether patients thought to be vegetative may harbor clinically undetectable interactivity.39 In one well-publicized case report that was published in Science in 200640 and subsequently disseminated by the lay press, researchers in the United Kingdom studied a patient who had been conclusively diagnosed clinically to be in a vegetative state five months after a traumatic brain injury and tested her brain with functional MRI studies. The researchers imaged her brain while reading her regular sentences, and also sentences that were non-sensical but acoustically similar to regular ones. Unexpectedly for a vegetative patient, the imaging showed a response in areas subserving auditory processing and comprehension in patterns similar to healthy volunteers. Acknowledging that these images did not demonstrate actual awareness, the investigators then scanned the patient's brain using fMRI while verbally instructing her to perform two imaginary tasks (playing tennis and walking through her house) during which her brain activation pattern not only resembled that of normal controls, but changed with the task requested, suggesting she was able to understand and respond with her thoughts, at least for the 30 seconds required for the scan.
This report was limited by involving only one patient, who was later thought to exhibit signs of persistent awareness, more consistent with the minimally conscious state, so it is possible that she was in a minimally conscious state at the time of the study, or that she fluctuated between a vegetative and minimally conscious state, or that she was one of the uncommon patients who emerge from a vegetative to a minimally conscious state within 12 months after a traumatic brain injury. The authors of the report were circumspect in their interpretation of these data. Despite that, an accompanying editorial cited a "rich mental life" for this patient.41
Although questions remain about the clinical condition of this patient prior to scan, the finding of unexpected interactions visible only on functional imaging has led to some speculation that in the future, the diagnostic criteria for the vegetative and minimally conscious states might someday be revised to include imaging results. Further, one can imagine that if more such cases are documented, technology could someday be used to help such patients communicate.
4.1.3. Prognostication and the notion of late recovery
Accuracy of diagnosis is important but becomes more urgent when coupled with the question of prognosis. Thus, the second major area of neuroimaging investigation pertinent to disorders of consciousness is that of prognostication and the notion of late recovery from the vegetative or minimally conscious state.
This issue gained attention in 2003 with the case of Terry Wallis, who in 1984 suffered a traumatic brain injury, and resided in an Arkansas nursing facility with the diagnosis of vegetative state. After 19 such years, without being seen by a neurologist or undergoing any neuroimaging, he unexpectedly regained speech in 2003.
This in turn leads to the question of predicting which patients are likely to eventually recover. At present, no study has validated a predictive model, but efforts to form one continue. A 2007 study by Di et al. used fMRI to study seven vegetative patients and four minimally conscious ones while the patients' own names were read to them by a familiar voice.42 Two of the clinically vegetative patients unexpectedly showed activation not only of auditory cortex, but of temporal and association areas, suggesting the structural possibility of higher thought; these two later went on to improve to minimal consciousness. Other investigators are using fMRI to examine patients already minimally conscious whose brains may have the structural integrity needed eventually to emerge to sustained awareness. The goal of these experiments is to devise individualized assessments, similar to those used to identify patients appropriate for neurosurgery43 to treat epilepsy, which will help evaluate whether patients have the potential for recovery from minimal consciousness.
Because of the lack of radiographic diagnostic, outcome, or prognostic data, there is no current recommendation, for example, to use fMRI or PET scanning as a screening tool for patients in either a vegetative or minimally conscious state. At present both are still mostly research techniques. Patients are currently being enrolled in trials to elucidate these points and recommendations may change as results of these studies emerge. If there has been a noticeable change in practice resulting from the recent reports of unexpected radiographic evidence of neural activity, especially auditory, it is probably clinicians' increased sensitivity and discretion regarding what is said at the patient's bedside. Increasingly, minimally conscious patients in particular are given the benefit of the doubt in terms of their capacity to hear and understand.
Functional neuroimaging offers a fresh, increasingly concrete interpretation of "meaning." These techniques now permit visualization of the level of activity of the brain structures thought responsible for the human ability to have thoughts and to synthesize meaning itself from sensory experience. Scans of vegetative patients showing destroyed connections between their receptive and processing areas support the idea that vegetative patients are disconnected from the parts of their brains which integrate sensory experience into meaning.44
4.1.4. Limitations of Imaging
As appealing and persuasive as images can be in distinguishing among states of consciousness, they have important scientific and technical limitations. Experts still dispute which areas of the brain are responsible for higher consciousness. Sedating medications to suppress unwanted movement and reduce artifact may be required for scanning. Sedation can certainly affect -- and often suppress -- brain activity, as can other routine medications (such as anticonvulsants) that patients may be receiving. Patients with abnormal electrical activity from epileptic or epileptogenic foci (often subclinical) may have increased metabolic activity in cortical areas reflecting pathology, rather than intentional use. For some patients, the presence of surgical scars or otherwise altered anatomy from, for example, hydrocephalus, makes the scans harder to interpret, much less compare, to a composite standard control.
Further, little is known about what threshold is required for observed metabolic activity or blood flow to translate into actual cognition. Even less is known about the quality of any cognition that might exist. Hirsch showed that a greater stimulus is required to obtain a response in fMRI of minimally conscious patients, but it is not known whether the signal observed is subjectively similar to that experienced by unimpaired patients.45,46
4.1.5. Research, Imaging, and Disorders of Consciousness
At the research level, functional and metabolic neuroimaging are enhancing scientists' understanding of states of consciousness. Technologies such as PET can provide information about which brain regions are affected after brain injury. Metabolic studies help researchers better understand the physiologic state of injured brain tissue.
While there have not been many successful or reproducible examples of using functional neuroimaging to diagnose disorders of consciousness, the technology is on the cusp of doing so. Functional neuroimaging may offer new ways to assess a patient's status and to predict outcome, as well as lead to a model-based approach that could guide new therapies for the recovery of consciousness. Medical treatment to repair disorders of consciousness, however, remains more of a hope than an imminent prospect.47
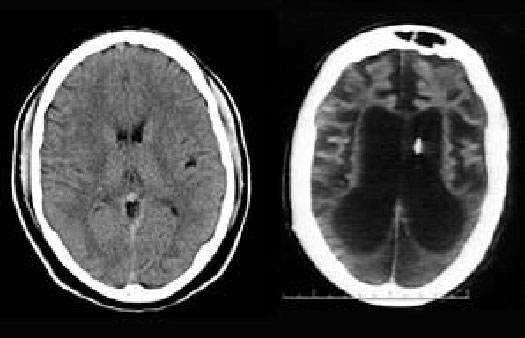
Source: Answers.com
4.2. Emerging Uses of Neuroimaging
Imaging technology is poised to contribute to neurology research in a number of ways. A 2003 meta-analysis, showed that in all fMRI studies with a clinical component conducted between 1991 and 2001, presurgical mapping studies accounted for 33% of the papers, major psychiatric disorders (including depression) accounted for 18%, and neurodegenerative diseases accounted for 12% of the studies. The remaining 37% of the studies looked at topics like drug and alcohol use, non-pathologic changes over the lifespan, and pediatric developmental delays.48 To demonstrate a sample practical application, Illes also asserts that neuroimaging techniques will transform the process of diagnosis, prediction, and clinical management of Alzheimer's disease (AD) and related dementias.49 While neuroimaging currently can be used only to exclude potential diagnoses, it is likely to play three important roles in the future. It may be used to increase the specificity in AD diagnoses, predict who is likely to develop AD, and replace standard outcome measures in therapeutic trials.
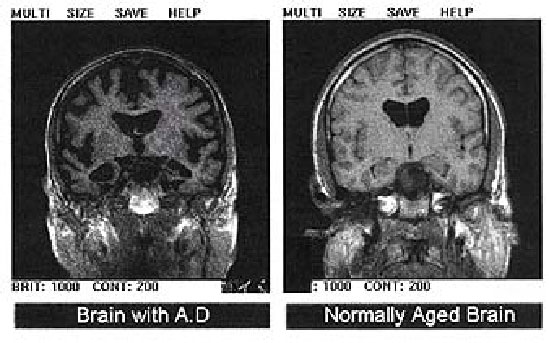
Source: Wikimedia
4.3. Non-medical uses of brain imaging
Imaging may also be used to conduct neurological research unrelated to disease by examining how the brain functions during different cognitive tasks. Our current understanding of decision-making has become even more complex due to the use of neuroimaging, as we have learned that high-cost and low-cost decision-making occur in entirely separate regions of the frontal lobes.50
The number of fMRI studies looking at decision-making tasks has increased within the last five years, not wholly because of greater availability of fMRI machines. The entire field of decision-making research is concentrating on questions of function at a systems level, rather than studying decision-making by examining analogous structures in simple model systems.51 Neuroimaging, therefore, is giving us more information about brain function as well as shaping the direction of the field.
4.3.1. Lie detection
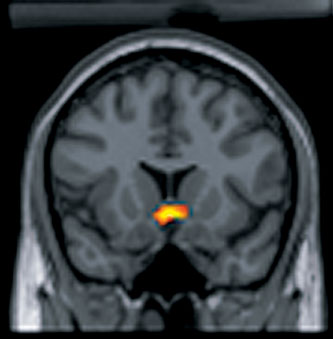
Source: Wikimedia
Careful neuroanatomical localization studies have combined with the latest functional neuroimaging techniques to facilitate localization of certain kinds of memory, intention and recognition--with implications being swiftly appropriated by the legal profession. Neuroimaging technology also has important implications for detecting false or mistaken memories, as opposed to outright deception, in witness testimonies.
The potential use of neuroimaging to detect lies has garnered a great deal of attention in the popular media.52,53,54 Cognitive neuroscience researchers have spent the last decade studying the neural systems we apparently use to lie or deceive, and have identified a number of brain regions that allow us to explicitly, consciously, and voluntarily recall memories. Clinical PET and fMRI studies consistently show that the anterior and inferior prefrontal regions of the cerebrum are activated during the intentional retrieval of information.55
As of now, fMRI is the neuroimaging technology most likely to be used in lie detection, based on the idea that lying requires more cognitive effort, and therefore more oxygenated blood, than truth telling, and so will be picked up by an fMRI scanner.56 Some foresee use of this technique as a modern form of polygraphy that directly, rather than indirectly, verifies truth and detects lies. Studies using fMRI have attempted to visualize the process of lying, with some success at showing relative activation of areas involved in self-control and restraint during experimentally controlled conscious deception.57
Despite published studies, many neuroscientists strongly question the results of these fMRI tests because many of the studies involve subjects who lie when instructed to do so, as opposed to lying wholly of their own volition.58 These two types of lying may in fact yield very different fMRI results, and consequently, much of the known data on lying may be relatively useless. To create a truly accurate study of lying, fMRI technology would have to be tested with people whose guilt or innocence has not yet been determined, but can be determined by other means after the scan. Finally, many assert that the current conception of neural activity during lying is far too simplistic to allow us to create true lie detection technology.59 It does not take into account different types of lies, such as "little white lies" or lies that are morally justified to protect someone. A sociopath may have a different conception of right and wrong, and so lying may evoke entirely different brain responses than the average individual.60 None of these variations has been seriously considered by those who claim to be developing lie-detection technology.
Unfortunately, the general public does not readily hear the reservations of these scientists, especially over the noise of entrepreneurs promoting their fMRI technologies for the purpose of lie-detection in the judicial system and in the security industry.61 As in the case of the polygraph, private industry is promoting the accuracy of a lie-detection device and encouraging companies, individuals, and even the state to adopt its use before its accuracy is assured. From an ethical perspective, this is an inappropriate use of the technology, as the false and inaccurate results that are likely to occur will provide an end-run around traditional methods of truth-seeking. Additionally, there is the fear that the use of such technology for these purposes will violate established notions of privacy.
4.3.2. Brain-typing / Personal Identity Traits
Neuroimaging is increasingly used by researchers to study the biological basis of personality. The types of information about personality traits that can be provided by brain imaging are fairly analogous to genetic information about personality, and include mental health vulnerabilities and predilections for violent crime, extroversion, neuroticism, risk-aversion, pessimism, persistence, and empathy, among others.62
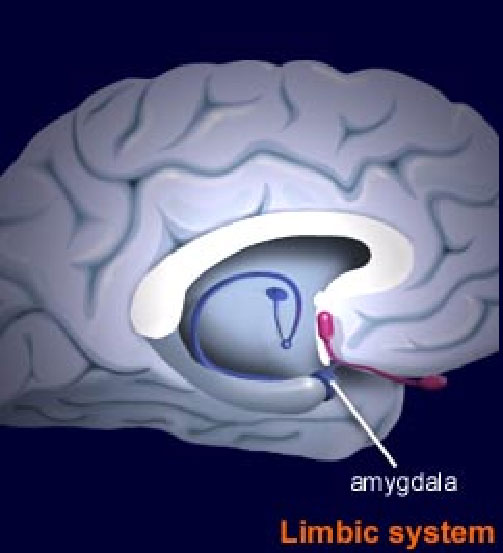
Source: Brainexplorer.org
Studies using fMRI have demonstrated that the amygdala, a structure known to play a role in emotional learning and evaluation, is activated differently when viewing faces with different skin tones.63,64 This may reflect subconscious racial biases, shaped by culturally acquired information and modified by individual experience. This may yet be a spurious conclusion, and we should be wary of branding people racists based on preliminary neuroimaging results. Another fMRI study demonstrated that in response to positive and negative stimuli, certain regions of the brain were activated to different degrees among a group of patients. The individual differences in activation corresponded to the two basic personality traits of extroversion and neuroticism.65
The use of brain imaging to predict personality traits is of little practical use if the same information can be obtained by more traditional personality surveys and tests. The important question, then, is whether brain imaging data can predict behavior better than traditional assessments of personality. To address this issue, Canli and colleagues used a series of fMRI experiments to show that neuroimages can, in fact, predict behavior better than either self-reported measures or reaction time data.66 They suggest that in the future, brain imaging data, combined with life history information and genetic information, may give us very precise predictors of behavior and personality.
Again, it is important to measure the value of such predictors. In our society, people are judged by their actions, not their thoughts. People overcome innate attributes through discipline, therapy, and will. There is a danger that using brain imaging technology to predict behavior and personality traits would negate the notion of free will and people's ability to act counter to their "nature."
4.3.3. Legal and forensic issues
Attempts to use scientific theories to exonerate defendants from responsibility for crimes date back centuries. In the 19th century, when social Darwinism was the sociological theory in vogue, criminals were viewed as moral degenerates partly condemned to their actions by failures in evolution. The advent of sophisticated neuroimaging techniques has transformed but not eliminated such arguments. If anything, it has dramatized them further.
Neuroimaging is increasingly used, although with much controversy, in the legal realm. In 1992, in what is regarded as the first court case that used neuroimaging to argue against a defendant's responsibility for his conduct, lawyers for Herbert Weinstein planned to show images of the defendant's abnormal arachnoid cyst.67 In reality, it was a benign and incidental finding having no relevance on why he might have killed and defenestrated his wife, but because of this potential "evidence," prosecutors accepted a plea bargain rather than risk the effect of the images on a jury. More recently, litigators have used functional imaging studies to argue that individuals lacked impulse control. Neuroscientific evidence of all sorts has been used within the last 15 years in court cases dealing with topics from head trauma to the effects of video games on aggression.
As with all scientific innovations used in jury trials, it is important to remember that we are asking non-scientists to interpret complex data that do not have easy explanations. Rather than understanding and offering judgment based on the science, these trials more frequently hinge on the persuasiveness of the arguments of the various expert witnesses.
In a development with science-fiction overtones, fMRI studies have attempted to visualize the process of lying, with some success at showing relative activation of areas involved in self-control and restraint during experimentally tightly controlled conscious deception.68 The studies are also being used to visualize general tendencies and predispositions. For example, some say that an overactive amygdala or an underactive prefrontal cortex renders their owners more prone to and/or less capable of resisting transgressive behaviors.69
Neuroscientists have emphasized that visualizing a metabolic tendency seen only with a highly sensitive, research-grade magnet is not tantamount to actual clinical expression of those tendencies, nor does it indicate that they are consistently present when the person is outside of the carefully controlled conditions of the scan. Scholars of both law and ethics have argued that even a pronounced tendency does not necessarily translate into action; that people should not discount the effect of reason over impulses or unconscious tendencies based on imaging data; and that free will and personal responsibility are still paramount, absent specific mitigating conditions of duress.
Arguments about these issues at the interface between neuroscience and law have spawned a new sub-discipline, Neurolaw.
5. Ethical issues
Eager to adopt any technology that will help patients, but cautious in deploying new technologies until they are supported by abundant and reproducible evidence, neurologists, and medicine as a whole, await the slow translation of neuroimaging research into clinical application. Somewhat strangely, techniques that have yet to find any clinical or medical utility are quickly gaining momentum in other settings. In courtrooms, classrooms and boardrooms around the world, uses of neuroimaging are exploding in number. The neuroethical implications of this rush to adopt unverified evidence of this work are immense.
5.1. Brain privacy
A futuristic concern is that imaging will one day advance to the point of ubiquity due to low cost and increased convenience, such that functional scanning may destroy the boundaries of privacy, allowing others--doctors, lawyers, marketers, the police, the government--to know what people are thinking. At least one scholar has asked rhetorically whether the brain may someday require privacy protections like homes that require a search warrant to enter.
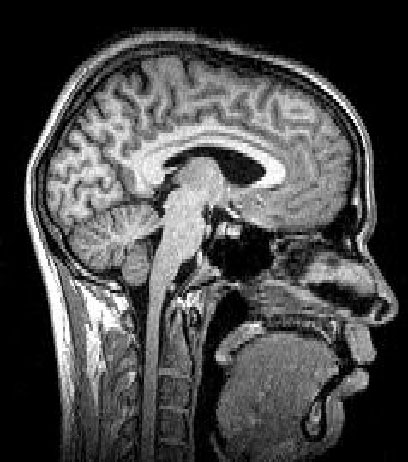
Source: Answers.com
In today's world, we must wonder if neuroimaging encroaches on the traditionally conceived zone of privacy that surrounds the self. Certainly, health insurance companies, life insurance companies, and prospective employers would likely want to use using neuroimaging to determine how "healthy" an individual's brain is, or to see how that brain would function in certain circumstances.70 This is similar to requests for genetic data, which has been a recent topic of controversy. Illes argues that brain data should certainly have as much, if not more, protection than genetic data.71 With the recent enactment of the Genetic Information Non-Discrimination Act of 2007, the U.S. government is moving toward protecting the genetic privacy of citizens.72 It is unclear, however, if this protection will eventually extend to neuroimaging as well, given how much less is known about the results and interpretation of brain imaging data.
We need to consider if an abnormality identified in a brain image would be considered a disability, and if this abnormality would then be protected under Title 1 of the Americans with Disabilities Act. The answer to this question would likely influence the levels of privacy afforded to imaging results, as well as the degrees of stigma attached to them.
The dilemma of brain privacy becomes especially acute when considering the applications of neuroimaging in the legal system, particularly when individuals testify as witnesses in court trials. Under the assumption (and this remains to be seen) that neuroimaging technology will become sophisticated enough to be used reliably as a method to detect deception, false memories, or mistaken memories, we must ask ourselves if an individual has the right to maintain the privacy of his or her choice to lie or tell the truth, to make bad judgments, or to relate false or flawed memories.
People may choose to remember something as more benign than it was, or as more caustic, but these memories and the ways they affect human experience are important for the ways they are remembered, rather than their accuracy. Some have suggested that the courts will have to decide if brain privacy is a right that is protected by the Constitution (potentially by the First, Fourth, and Fifth amendments).73 There is also an important distinction between deliberate lies and false memories.
5.2. Neuro-essentialism
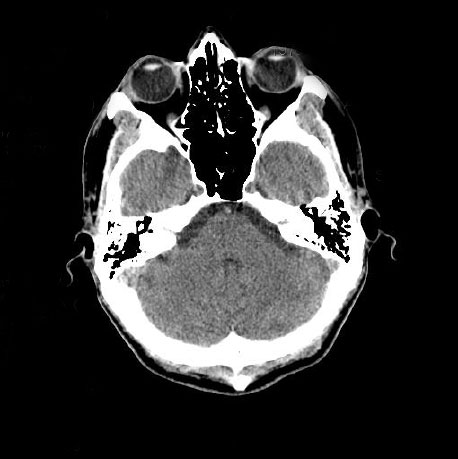
Source: Wikimedia
Neuro-essentialism is the notion that personal identity is not simply housed in the brain, but is the brain itself. This notion perpetuates an ill-informed and hasty reduction of identity to the brain. Although the distinction between the brain and the "self" is becoming increasingly fraught with complications,74 most would agree that there is a difference. When we say, "She is nice," we don't mean, "Her brain is nice."75
With the burgeoning use of neuroimaging technology, many neuroethicists are concerned by the dominance of neuro-essentialism as a mode of understanding selfhood and the brain in media depictions and popular opinions of neuroimaging. Neuro-essentialism also contributes to the widespread uneasiness often triggered by research into the biology of what makes us behave as we do. If neuroscientists deconstruct how subjective experience arises from neural computation, then we may lose a sense of what it means to be human,76 in that we are no longer able to choose our reactions to stimuli. Others similarly worry that we may have to accept that we are not free agents responsible for our own choices and behaviors altogether, resulting in a demystification of the human experience.
Many, however, assert that these notions of fatalism and demystification do not pose a valid concern, that neuroimaging cannot provide solid, incontrovertible evidence either for or against behavioral determinism77 and people will always need to consider influencing factors outside of one's own skull. Furthermore, new research on brain plasticity and neural reorganization after injury have suggested that a great deal of intervening external and cultural factors constantly change and influence us beyond our brain chemistry and physiology.78
5.3. Clinical Research Dilemmas
While many ethical dilemmas discussed here are predicated on the assumption that neuroimaging technology will evolve to even more spectacular and specific capabilities, we are more acutely challenged by significant ethical issues pertaining to the technology as it is now. Even as advances in neurology and cognitive psychology are published, current and traditional concerns with testing human subjects remain just as relevant and worthy of attention.
5.3.1. Recruitment and informed consent
Researchers who utilize neuroimaging technology have recognized the need to consider how the informed consent process can work for an individual with impaired capacity to consent, especially as many neurology studies are designed to recruit exactly that patient population. In addition, there are considerations with informed consent that are unique to neuroimaging, given that little is known about risks and benefits of neuroimaging, and what a neuroimage portends for a person's health.79
Significant challenges also arise from potential investigator bias in selecting study subjects, which may influence the direction of research. In autism research, for example, where the severity of the condition of potential subjects falls along a spectrum, investigators are more likely to recruit higher-functioning individuals as they are easier to work with in a neuroimaging context. They can stay still longer and can follow instructions more reliably. Consequently, both neuroimaging technology and the studies themselves increasingly may be oriented toward the higher-functioning population, neglecting the lower- functioning individuals.
5.3.2. Safety
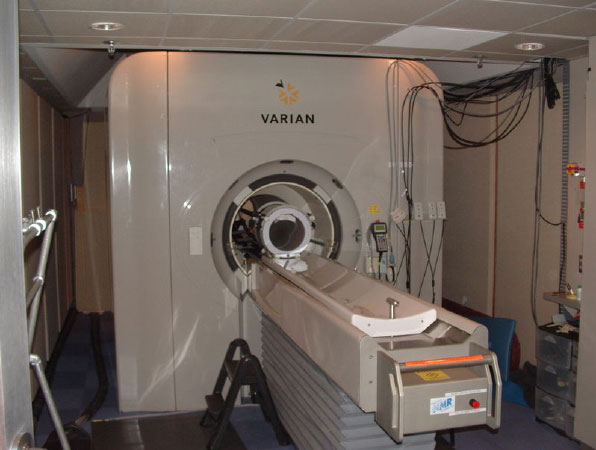
Source: Wikipedia
Little is known about the safety of some neuroimaging techniques. Currently, fMRI is valued over other imaging techniques such as PET and SPECT scans because it relies on non-invasive magnetic techniques, rather than exposure to radioactive substances injected into the bloodstream. Institutional Review Boards and Research Ethics Boards usually classify research with MRI as minimal- to moderate-risk, depending in part on the use of contrast agents, sedation, and the age and vulnerability of the population. Known risks for MRI include claustrophobia and sensitivities to particular stimuli. For subjects with metal implants, they risk their implants interfering with the image, as well as internal damage due to the pull of the MRI's strong magnetic fields. Alternatively, they may not be permitted to get an MRI, even though it is otherwise the most appropriate diagnostic tool. Additionally, certain risks are more speculative, such as the long-term effects of exposure to a powerful magnet. At the present time, given neither empirical nor anecdotal evidence to suggest any real risk, use of these techniques continues to grow.
5.3.3. Incidental discoveries of brain anomalies
A pervasive concern for neuroscientists who use brain imaging protocols is what to do when a subject is discovered to have some type of brain anomaly. If the individual is a control subject, then the anomaly is entirely unexpected. In the case of patients, the anomaly may be completely unrelated to the purpose of the imaging study. What then, should be done with this information? Should it be disclosed to the subject, when, and by whom? Answers to these questions should be addressed in the study protocol and in the informed consent process.
Incidental discoveries in neurology are not an uncommon phenomenon. Current data suggest that about 15% of asymptomatic research subjects in neuroimaging research protocols are found to have a brain anomaly, and the findings are clinically significant for 13-53% of these individuals.80 There is no consistent practice or mandated protocol to deal with this situation. Even in the informed consent process, it is hard to determine if a participant really understands the concept of "potential incidental finding," and freely chooses to opt in or out of disclosure.81 Furthermore, since neuroimaging technology has not been explored fully for the potential to test asymptomatic individuals, incidental findings are not supported by empirical evidence.
The dilemma of the incidental finding is not unique to neuroimaging, but neuroethicists are making early efforts to address this issue.82 Could knowledge of an incidental finding, in the wrong hands, lead to preemptive allocation of resources (by insurance companies) or opportunities (for jobs) or prospective surveillance (by police) or denial of benefits?
5.3.4. Data interpretation
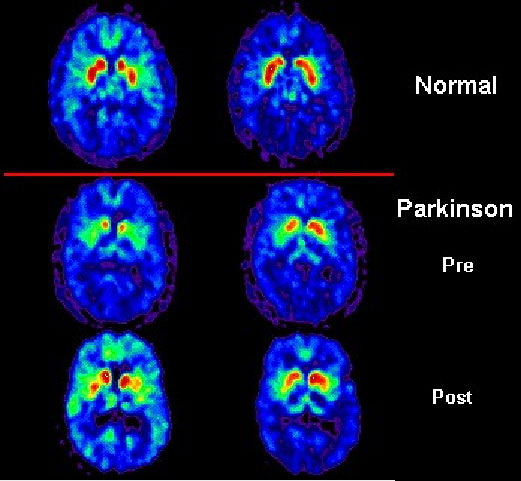
Source: Michigan State University
The sheer complexity of neuroimaging research makes the acquisition and meaningful interpretation of data in neuroimaging studies daunting to neuroscientists and neuroethicists alike. It is imperative to remember that neuroimages are not photographs. They are not "snapshots" of the brain, and there are a number of inferential steps between the brain and the raw data, and between the raw data and the published image.83 The public often does not know or understand that the majority of published images represent an aggregation of data, meaning the combination of the results from many individual brain scans. Therefore, little can be known about the significance of differences between individuals.84
Furthermore, many question the ability of investigators to interpret brain images objectively. Researchers themselves often cite concerns with getting accurate, reproducible images, and either over-interpret or misinterpret data dealing with neurological artifacts. Managing to convey the results accurately to both the lay and scientific public can be an elusive goal.85 An undeniably large amount of variation in research designs, statistical treatment of the data, and image resolution add complexity to accurate and reproducible data interpretation.
5.4. Public opinion and knowledge of neuroimaging
Another significant risk arising from the growing use of neuroimaging is the phenomenon of neuro-realism: when a mental or physical phenomenon is studied with advanced technology, people tend to assume the phenomenon is real, without any regard to actual study results.86 This certainly is true of neuroimaging: the public tends to view brain scans as more accurate and objective than they really are.87 We emphasize that one of the most important responsibilities for both neuroethicists and neuroimaging researchers is to ensure that neuroimaging results and data are accurately conveyed to the public, along with all the related imperfections and uncertainty.
It seems reasonable to assume that we find neuroimages to be so convincing (regardless of context or legitimacy) because of the way we have culturally defined and invested the brain with meaning as the seat of the mind and the self.88 As visual animals, we find a picture or an image compelling, and we have a heritage of seeing pictures as truth. It is important to remember that in the case of neuroimaging, a pictoral representation is not a guarantee of accuracy.
5.5. Misappropriation of neuroimaging technology
The lay public, via the popular and journalistic media, are not the only ones who either intentionally or unintentionally misuse neuroimaging. The corporate world eagerly anticipates the point at which it can use neuroimaging for the purposes of "neuro-marketing," where brain imaging could be used to measure the consumer's desire for a product and subsequently, to shape an effective marketing campaign.89,90 Furthermore, a growing group of entrepreneurs intends to market neuroimaging directly to consumers. One example finds companies trying to develop portable, flexible fMRIs for employers to screen potential employees.91 Other uses include the attempt to outsource lie-detection technology, either to companies to test their employee's loyalty, or to spouses to test their partner's fidelity.92
Little can be said for the accuracy or appropriateness of these uses. We simply do not have the temporal or spatial resolution to make definitive statements about such issues. Most importantly, we don't know if higher brain function that reflects complex human qualities can be localized to one or a few brain regions. Neural correlates of loyalty, for example, may be distributed in thousands of complex neural networks that cannot be captured with current techniques.
The medical community is not entirely innocent of the misrepresentation of imaging technologies. While controversial, there is an increasing use of full body CT scans being marketed directly to the public by private practice doctors to discover any hints of developing ailments.93 These scans are extremely expensive, yield questionable results, and entirely change the way people can be reassured that they are healthy. People have argued that this technology causes undue worry in otherwise healthy people, while it encourages a less vigilant approach to those with poor health habits. There is a danger that neuroimaging technology will be similarly marketed to the public who seek to know more about their brains and risks of brain diseases.
While "direct-to-consumer" neuroimaging entrepreneurs are increasingly becoming a reality, neuro-marketing remains an improbable future. In contemplating the ethical implications of neuroimaging, one must consider not only the present reality, but, equally important, we must consider the obligations of neuroimaging researchers for the future. Are they responsible for the legitimate or nefarious future uses to which neuroimaging might be put? Is there a special commitment to the consumer in terms of follow up? Are these brain images considered private "data," and if so, how are they protected? These are urgent questions for the entire field of neuroimaging and neuroethics to address.94
6. Neuroimaging Technology in Popular Culture
6.1. The Truth Machine
The Truth Machine, a 1996 science fiction novel by James L. Halperin, features a man who invents an infallible lie detector, and consequently changes the world. Citizens are required to pass lie detector tests in order to gain a driver's license, have children, move to a different state, get hired for a job, and so on. Eventually, a small and imperceptible version is developed, and people begin wearing them all the time, thus eliminating dishonesty, crime, terrorism and a great deal of general social problems. The book focuses on the effects on society and humanity of people being unable to lie and of people eventually forgetting that lying is an option. Halperin paints a utopian picture of this hypothetical society. For example, there are no erroneous executions of innocent people. However, despite Halperin's world view, it is worthwhile to consider some of the negative consequences that ubiquitous lie detection technology might have for society, especially given how many researchers and engineers aim to market lie detection technology (of dubious quality) to consumers, employers, and the government.
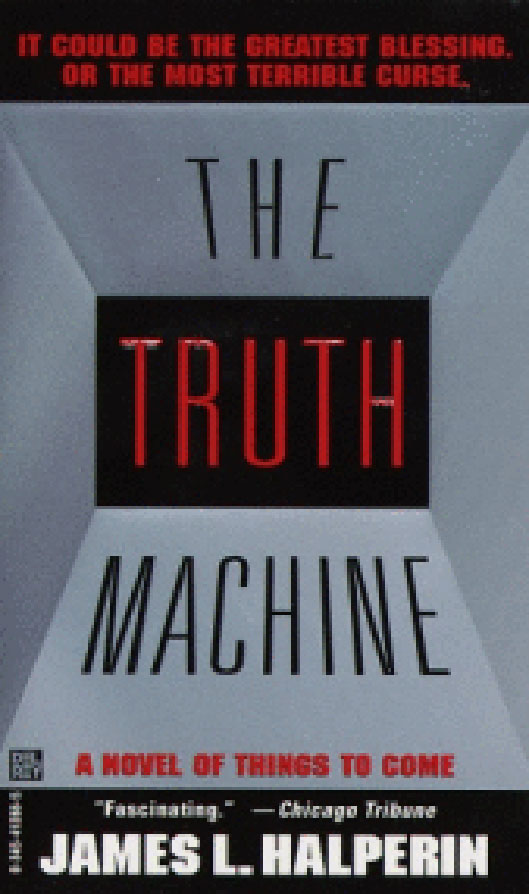
Source: Random House Publishing
6.2. Minority Report
A 2002 science-fiction film loosely based on a novel written by Phillip K. Dick in 1956, Minority Report is a vision of the future in which criminals can be caught before they even commit a crime. Three psychics, or "precogs" comprise the "technology" that makes this possible, as they can sense when someone is about to commit a murder. The pre-cogs translate that premonition into images and information that can lead the Department of Pre-Crime, a high-tech policing division, to the potential criminal before the criminal act. The movie revolves around one of the officers in the Department of Pre-Crime who is accused of an intended murder, and sets out to prove his innocence. In doing so, he reveals the fatal flaw in this pre-crime system: if people know their own futures, they can choose to change them. Although the "pre-cogs" are simply a science fiction device, many researchers and scientists anticipate using neuroimaging technology to identify people pre-disposed to crime, or even to anticipate what kinds of actions they may be planning. This could create a society where people are routinely scanned for pre-meditative violent or criminal tendencies.
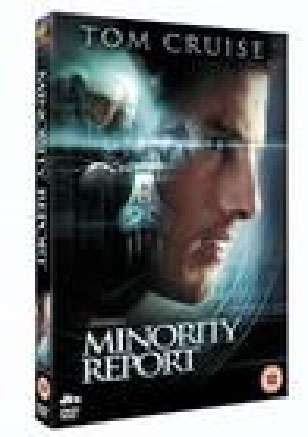
Source: IMDB.com
Please click here to go to the interesting cases and challenging questions.
1Raichle M. Neuroimaging. The Dana Alliance for Brain Initiatives. The 2006 Progress Report
on Brain Research. New York: Dana Press; 2006.
2 Kevles BH. Naked to the Bone. Reading, MA: Perseus Books Group; 1998, p. 101.
3 Hyman, SE. Neuroethics: At Age 5, the Field Continues to Evolve. The 2007 Progress Report
on Brain Research. New York: Dana Press; 2007, p. 9.
4 The 2007 Progress Report on Brain Research. New York: Dana Press; 2007, p. 35.
5 Illes J, Racine E. Imaging or imagining? A neuroethics challenge informed by genetics. Am J
Bioeth. 2005 Spring; 5(2): 5-18.
6 Illes J, Racine E, Kirschen MP. A Picture is worth 1000 words, but which 1000? Neuroethics:
Defining the Issues in Theory, Practice, and Policy (J Illes, ed.). New York: Oxford University
Press; 2006. p. 151.
7 Medline Plus [Web site on the Internet]. EEG. [updated 2006 Sept 25; cited 2007 Aug 14].
Available from: http://www.epilepsy.com/epilepsy/meg_intro.html.
8 Illes J, Racine E, Kirschen MP. A Picture is worth 1000 words, but which 1000? Neuroethics:
Defining the Issues in Theory, Practice, and Policy (J Illes, ed.). New York: Oxford University
Press; 2006. p. 151.
9 Medline Plus [Web site on the Internet]. EEG. [updated 2006 Sept 25; cited 2007 Aug 14].
Available from: http://www.epilepsy.com/epilepsy/meg_intro.html.
10 Martinos Center for Biomedical Imaging [Web site on the Internet]. Imaging Technologies:
Magnetoencephalography (MEG). [updated 2004 Dec; cited 2007 Aug 14]. Available from:
http://www.nmr.mgh.harvard.edu/martinos/research/technologiesMEG.php.
11 Department of Health and Human Services. What's NEXT? Nationwide Evaluation of X-ray
Trends: 2000 computed tomography. (CRCPD publication no. NEXT_2000CT-T.) Conference
of Radiation Control Program Directors, 2006. Available at
http://www.crcpd.org/Pubs/NextTrifolds/NEXT2000CT_T.pdf.
12 Brenner, DJ and Hall, EJ. Computed Tomography - An Increasing Source of Radiation
Exposure. N Engl J Med 2007;357:2277-84.
13 Brenner, DJ and Hall, EJ. Computed Tomography - An Increasing Source of Radiation
Exposure. N Engl J Med 2007;357:2277-84.
14 The Cleveland Clinic [Web site on the Internet]. PET scan. [cited 2007 Aug 14]. Available
from: http://www.clevelandclinic.org/health/health-info/docs/3400/3462.asp?index=10123.
15 Ackerman, SJ. Hard Science, Hard Choices: Facts, Ethics, and Policies Guiding Brain
Science Today, 2006. New York: Dana Press; 2006, p. 26.
16 PETNET Solution [Web site on the Internet]. Positron Emission Tomography (PET). [cited
2007 Aug 14]. Available from: http://www.petscaninfo.com/zportal/portals/pat/basic.
17 Illes J, Racine E, Kirschen MP. A Picture is worth 1000 words, but which 1000? Neuroethics:
Defining the Issues in Theory, Practice, and Policy (J Illes, ed.). New York: Oxford University
Press; 2006. p. 151.
18 The Cleveland Clinic [Web site on the Internet]. PET scan. [cited 2007 Aug 14]. Available
from: http://www.clevelandclinic.org/health/health-info/docs/3400/3462.asp?index=10123.
19 Illes J, Racine E, Kirschen MP. A Picture is worth 1000 words, but which 1000? Neuroethics:
Defining the Issues in Theory, Practice, and Policy (J Illes, ed.). New York: Oxford University
Press; 2006. p. 151.
20 Brain Matters [Web site on the Internet]. Brain Scan: SPECT Imaging. [cited 2007 Aug 14].
Available from: http://www.brainmattersinc.com/spect.html.
21 Amen Clinic, Inc. [Web site on the Internet]. Brain SPECT Imaging. [cited 2007 Aug 14].
Available from: http://amenclinics.com/bp/atlas/ch1.php.
22 Illes J, Racine E, Kirschen MP. A Picture is worth 1000 words, but which 1000? Neuroethics:
Defining the Issues in Theory, Practice, and Policy (J Illes, ed.). New York: Oxford University
Press; 2006. p. 151.
23 Amen Clinic, Inc. [Web site on the Internet]. Brain SPECT Imaging. [cited 2007 Aug 14].
Available from: http://amenclinics.com/bp/atlas/ch1.php.
24 FMRIB Centre, University of Oxford [Web site on the Internet]. Introduction to fMRI-
Background. [cited 2007 Aug 15]. Available from:
http://www.fmrib.ox.ac.uk/education/fmri/introduction-to-fmri/background.
25 Columbia fMRI [Web site on the Internet]. The Future Role of functional MRI in Medical
Applications. [cited 2007 Aug 15]. Available from: http://www.fmri.org/fmri.htm.
26 fMRI for Newbies [Web site on the Internet]. fMRI for Newbies: A crash course in brain
imaging. [cired 2007 Aug 21]. Available from: http://psychology.uwo.ca/fmri4newbies/.
27 Columbia fMRI [Web site on the Internet]. The Future Role of functional MRIin Medical
Applications. [cited 2007 Aug 15]. Available from: http://www.fmri.org/fmri.htm.
28 FMRIB Centre, University of Oxford [Web site on the Internet]. Introduction to fMRI- Clinical
and Commercial Use. [cited 2007 Aug 15]. Available from:
http://www.fmrib.ox.ac.uk/education/fmri/introduction-to-fmri/clinical-commercial-use/.
29 FMRIB Centre, University of Oxford [Web site on the Internet]. Introduction to fMRI-
Background. [cited 2007 Aug 15]. Available from:
http://www.fmrib.ox.ac.uk/education/fmri/introduction-to-fmri/background.
30 Illes J, Racine E. Imaging or imagining? A neuroethics challenge informed by genetics. Am J
Bioeth. 2005 Spring; 5(2): 5-18.
31 The Multi-Society Task Force on PVS. Medical Aspects of the Persistent Vegetative State-
Second of Two Parts. N Engl J Med. 1994; 330: 1499-1508.
32 The Multi-Society Task Force on PVS. Medical Aspects of the Persistent Vegetative State-
Second of Two Parts. N Engl J Med. 1994; 330: 1572-1579.
33 Hirsch J. Raising consciousness. J Clin Invest. 2005; 115: 1102.
34 McCullagh P. Consciousness in a Vegetative State? A Critique of the PVS Concept. London:
Kluwer Academic Publishers; 2004.
35 The Hypothermia after Cardiac Arrest Study Group. Mild Therapeutic Hypothermia to
Improve the Neurologic Outcome after Cardiac Arrest. N Engl J Med. 2002; 346:549-556.
36 Laureys S, Owen AM, Schiff ND. Brain function in coma, vegetative state, and related
disorders. The Lancet Neurology. 2004 Sept; 3(9): 537-546.
37 Schiff ND, Rodriguez-Moreno D, Kamal A, Kim KHS, Giacino JT, Plum F, Hirsch J. fMRI
reveals large-scale network activation in minimally conscious patients. Neurology. 2005; 64:
514-523.
38 Bernat JL. Chronic disorders of consciousness. The Lancet. 2006; 367(9517): 1181-1193.
39 Owen AM, Coleman MR, Boly M, Davis MH, Laureys S, Pickard JD. Using functional
magnetic resonance imaging to detect covert awareness in the vegetative state. Arch Neurol.
2007 Aug; 64(8): 1098-102.
40 Owen AM, Coleman MR, Boly M, Davis MH, Laureys S, Pickard JD. Detecting Awareness in
the Vegetative State. Science. 2006 Sept 8; 313(5792): 1402.
41 Naccache L. Psychology: Is She Conscious? Science. 2006 Sept 8; 313(5792):1395-6.
42 Di HB, Yu SM, et al. Cerebral response to patient's own name in the vegetative and minimally
conscious states. Neurology. 2007; 68: 895-899.
43 Hirsch J, Ruge MI, Kim KHS, Correa DD, Victor JD, Relkin NR, Labar DR, Krol G, Bilsky MH,
Souwedane MM, DeAngelis LM, Gutin PH. An Integrated Functional Magnetic Resonance
Imaging Procedure for Preoperative Mapping of Cortical Areas Associated with Tactile,
Motor, Language, and Visual Functions. Neurosurgery. 2000; 47: 711-722.
44 Laureys S, Owen AM, Schiff ND. Brain function in coma, vegetative state, and related
disorders. The Lancet Neurology. 2004 Sept; 3(9): 537-546.
45 Hirsch J. Raising consciousness. J Clin Invest. 2005; 115: 1102.
46 Giacino JT, Hirsch J, Schiff N, Laureys S. Functional Neuroimaging Applications for
Assessment and Rehabilitation Planning in Patients With Disorders of Consciousness.
Archives of Physical Medicine and Rehabilitation. 2006 Dec; 87 (12; Supplement 1): 67-76.
47 Ackerman SJ. Hard Science, Hard Choices: Facts, Ethics, and Policies Guiding Brain
Science Today, 2006. New York: Dana Press; 2006, p. 42.
48 Illes J, Kirschen M. New Prospects and Ethical Challenges for Neuroimaging Within and
Outside the Health Care System. Am J Neuroradiol. 2003 Nov-Dec; 24(10): 1932-4.
49 Illes J, Rosen A, Greicius M, Racine E. Prospects for prediction: ethics analysis of
neuroimaging in Alzheimer's disease. Annals NY Acad Sci. 2007 Feb; 1097: 278-95.
50 Volz KG, Schubotz RI, von Cramon DY. Decision-making and the frontal lobes. Current
Opinion in Neurol. 2006 Aug; 19(4): 401-6.
51 Balleine BW. The Neural Basis of Choice and Decision Making. J Neurosci. 2007 Aug 1;
27(31): 8159-8160. Available from: http://www.jneurosci.org/cgi/content/short/27/31/8159.
52 Rosen J. The Brain on the Stand. New York Times Magazine. 2007 March 11. Available
from:
http://www.nytimes.com/2007/03/11/magazine/11Neurolaw.t.html?pagewanted=1&ei=5088...
&en=bbdc98f5f3800cd7&ex=1331269200.
53 Racine E, Bar-Ilan O, Illes J. Brain Imaging: A decade of coverage in the print media. Sci
Commun. 2006 Sep; 28(1): 122-142.
54 Talbot, M. Duped: can brain scans uncover lies? The New Yorker. 2007 Jul 2, pp. 52-61.
Available from: http://www.newyorker.com/reporting/2007/07/02/070702fa_fact_talbot.
55 Illes, J. A fish story? Brain maps, lie detection, and personhood. Cerebrum. 2004 Fall;
6(4):73-80. Available from: http://www.dana.org/news/cerebrum/detail.aspx?id=1200.
56 Talbot, M. Duped: can brain scans uncover lies? The New Yorker. 2007 Jul 2, pp. 52-61.
Available from: http://www.newyorker.com/reporting/2007/07/02/070702fa_fact_talbot.
57 Illes, J. A fish story? Brain maps, lie detection, and personhood. Cerebrum. 2004 Fall;
6(4):73-80. Available from: http://www.dana.org/news/cerebrum/detail.aspx?id=1200.
58 Illes, J. A fish story? Brain maps, lie detection, and personhood. Cerebrum. 2004 Fall;
6(4):73-80. Available from: http://www.dana.org/news/cerebrum/detail.aspx?id=1200.
59 Ackerman SJ. Hard Science, Hard Choices: Facts, Ethics, and Policies Guiding Brain
Science Today, 2006. New York: Dana Press; 2006, p. 34.
60 Fischbach RL, Fischbach GD. The brain doesn't lie. Am J Bioeth. 2005 Spring; 5(2): 54-5.
61 Silberman S. Don't Even Think About Lying: How brain scans are reinventing the science of
lie detection. Wired. [cited 2007 Sept 7]. Available from:
http://www.wired.com/wired/archive/14.01/lying_pr.html.
62 Farah M. Neuroethics: A Guide For The Perplexed. Cerebrum. 2004; 6(4): 32. Available
from: http://www.dana.org/news/cerebrum/detail.aspx?id=1080.
63 Phelps EA, O'Connor KJ, Cunningham WA, Funayama ES, Gatenby JC, Gore JC, Banaji
MR. Performance on indirect measures of race evaluation predicts amygdala activation. J
Cogn Neurosci. 2000 Sep; 12(5): 729-38.
64 Ronquillo J, Denson TF, Lickel B, Lu ZL, Nandy A, Maddox KB. The effects of skin tone on
race-related amygdala activity: an fMRI investigation. Social Cognitive and Affective
Neuroscience. 2007; 2(1): 39-44.
65 Canli T, Zhao Z, Desmond JE, Kang E, Gross J, Gabrieli JD. An fMRI study of personality
influences on brain reactivity to emotional stimuli. Behav Neurosci. 2001 Feb; 115(1): 33-42.
66 Canli T. When genes and brains unite: ethical implications of genoming neuroimaging.
Neuroethics: Defining the Issues in Theory, Practice, and Policy (J Illes, ed.). New York:
Oxford University Press; 2006, p. 175.
67 Rosen J. The Brain on the Stand. New York Times Magazine. 2007 March 11. Available
from:
http://www.nytimes.com/2007/03/11/magazine/11Neurolaw.t.html?pagewanted=1&ei=5088...
&en=bbdc98f5f3800cd7&ex=1331269200.
68 Illes, J. A fish story? Brain maps, lie detection, and personhood. Cerebrum. 2004 Fall;
6(4):73-80. Available from: http://www.dana.org/news/cerebrum/detail.aspx?id=1200.
69 Pridmore S, Chambers A, McArthur M. Neuroimaging in psychopathy. Australian & New
Zealand Journal of Psychiatry. 2005 Oct; 39(10): 856-865.
70 Ackerman SJ. Hard Science, Hard Choices: Facts, Ethics, and Policies Guiding Brain
Science Today, 2006. New York: Dana Press; 2006, p. 29.
71 Illes J, Racine E. Imaging or imagining? A neuroethics challenge informed by genetics. Am J
Bioeth. 2005 Spring; 5(2): 5-18.
72 National Human Genome Research Institute [Web site on the Internet]. Genetic Information
Nondiscrimination Act of 2007. [cited 2007 August 20]. Available from:
http://www.genome.gov/24519851.
73 Ackerman SJ. Hard Science, Hard Choices: Facts, Ethics, and Policies Guiding Brain
Science Today, 2006. New York: Dana Press; 2006, p. 30.
74 Pinker S. The Mystery of Consciousness. Time. 2007 Jan 29; 169(5): 58-70. Available from:
http://www.time.com/time/magazine/article/0,9171,1580394,00.html.
75 Ackerman SJ. Hard Science, Hard Choices: Facts, Ethics, and Policies Guiding Brain
Science Today, 2006. New York: Dana Press; 2006, p. 36.
76 Pinker S. The Mystery of Consciousness. Time. 2007 Jan 29; 169(5): 58-70. Available from:
http://www.time.com/time/magazine/article/0,9171,1580394,00.html.
77 Roskies A. Everyday Neuromorality. Cerebrum. 2004 Fall; 6(4): 58-65. Available from:
http://www.dana.org/news/cerebrum/detail.aspx?id=1090.
78 Illes J, Racine E. Imaging or imagining? A neuroethics challenge informed by genetics. Am J
Bioeth. 2005 Spring; 5(2): 5-18.
79 Illes J, De Vries R, Cho MK, Schraedley-Desmond P. ELSI priorities for brain imaging. Am J
Bioeth. 2006 March; 6(2): W24-31.
80 Illes J. 'Pandora's box' of incidental findings in brain imaging research. Nat Clin Pract Neurol.
2006 Feb; 2(2): 60-1.
81 Illes J, Kirschen MP, Edwards E, Stanford LR, Bandettini P, Cho MK, Ford PJ, Glover GH,
Kulynych J, Macklin R, Michael DB, Wolf SM. Ethics: Incidental findings in brain imaging
research. Science. 2006 Feb 10; 311(5762): 783-4.
82 National Institutes of Health and Stanford University [Web site on the Internet]. Detection and
disclosure of incidental findings in neuroimaging research. [cited 2007 Aug 20]. Available
from: www.ninds.nih.gov/news_and_events/proceedings/ifexecsummary.htm.
83 Roskies A. Everyday Neuromorality. Cerebrum. 2004 Fall; 6(4): 58-65. Available from:
http://www.dana.org/news/cerebrum/detail.aspx?id=1090.
84 Ackerman SJ. Hard Science, Hard Choices: Facts, Ethics, and Policies Guiding Brain
Science Today, 2006. New York: Dana Press; 2006, p. 26.
85 Illes J, De Vries R, Cho MK, Schraedley-Desmond P. ELSI priorities for brain imaging. Am J
Bioeth. 2006 March; 6(2): W24-31.
86 Ackerman SJ. Hard Science, Hard Choices: Facts, Ethics, and Policies Guiding Brain
Science Today, 2006. New York: Dana Press; 2006, p. 36.
87 Farah M. Neuroethics: A Guide For The Perplexed. Cerebrum. 2004; 6(4): 32. Available
from: http://www.dana.org/news/cerebrum/detail.aspx?id=1080.
88 Illes J, Racine E, Kirschen MP. A Picture is worth 1000 words, but which 1000? Neuroethics:
Defining the Issues in Theory, Practice, and Policy (J Illes, ed.). New York: Oxford University
Press; 2006. p. 161.
89 Farah M. Neuroethics: A Guide For The Perplexed. Cerebrum. 2004; 6(4): 36. Available
from: http://www.dana.org/news/cerebrum/detail.aspx?id=1080.
90 Racine E, Bar-Ilan O, Illes J. Brain Imaging: A decade of coverage in the print media. Sci
Commun. 2006 Sep; 28(1): 122-142.
91 Racine E, Bar-Ilan O, Illes J. Brain Imaging: A decade of coverage in the print media. Sci
Commun. 2006 Sep; 28(1): 122-142.
92 Talbot, M. Duped: can brain scans uncover lies? The New Yorker. 2007 Jul 2, pp. 52-61.
Available from: http://www.newyorker.com/reporting/2007/07/02/070702fa_fact_talbot.
93 Bowes P. US Doctors offer full body scan. BBC News. 2001 Jan 2. Available from:
http://news.bbc.co.uk/1/hi/health/1097136.stm.
94 Illes J, Racine E, Kirschen MP. A Picture is worth 1000 words, but which 1000? Neuroethics:
Defining the Issues in Theory, Practice, and Policy (J Illes, ed.). New York: Oxford University
Press; 2006. p. 163.
95 Jennett B, Bond M. Assessment of outcome after severe brain damage. Lancet. 1975; 1,
480-484.
96 Jennett B, Bond M. Assessment of outcome after severe brain damage. Lancet. 1975; 1,
480-484.
97 Brain Trauma Foundation. Management and prognosis of severe traumatic brain injury.
American association of neurological surgeons. 2001.
References for Sidebars
S1 Wikipedia, The Free Encyclopedia [Web site on the Internet]. Pneumoencephalography.
[cited 2007 August 15]. Available from: http://en.wikipedia.org/wiki/Pneumoencephalography.
S2 Lee CI, Haims AH, Monico EP, Brink JA, Forman HP. Diagnostic CT scans: assessment of
patient, physician, and radiologist awareness of radiation dose and possible risks. Radiology
2004;231:393-398.
S3 Illes J, Racine E, Kirschen MP. A Picture is worth 1000 words, but which 1000? Neuroethics:
Defining the Issues in Theory, Practice, and Policy (J Illes, ed.). New York: Oxford University
Press; 2006, p. 151.
S4 Wikipedia, The Free Encyclopedia [Web site on the Internet] Minimally Conscious State.
[cited 2007 August 15]. Available from:
http://en.wikipedia.org/wiki/Minimally_conscious_state.
S5 Preston R. An Error in the Code: What can a rare disorder tell us about human behavior? The
New Yorker. 2007 August 13, p. 30.
S6 Eaton ML, Illes J. Commercializing cognitive neurotechnology- the ethical terrain. Nat
Biotechnol. 2007 Apr; 25(4): 393-7.
S7 Jenett B, Teasdale G, Braakman R, et al. Prognosis of patients with severe head injury.
Neurosurgery. 1979; 4: 283-289.
S8 Levy DE, Bates D, Caronna JJ, et al. Prognosis in nontraumatic coma. Ann Intern Med. 1981;
94: 293-301.
S9 Posner JB, Saper CB, Schiff N, Plum F. Plum and Posner's Diagnosis of Stupor and Coma.
USA: Oxford University Press; 2007.